CD34
2013-08-06
: Pdf Cd34 CD34 8 2013 pdf
Open the PDF directly: View PDF .
Page Count: 9

263Oral & Craniofacial Tissue Engineering
Translational Research: The CD34+ Cell Is Crucial
for Large-Volume Bone Regeneration from the
Milieu of Bone Marrow Progenitor Cells in
Craniomandibular Reconstruction
Robert E. Marx, DDS1/David B. Harrell, PhD, OF, FRIPH2
Purpose: This study investigated the role of the bone marrow–derived CD34+ cell in a milieu of
osteoprogenitor cells, bone marrow plasma cell adhesion molecules, recombinant human bone
morphogenetic protein (rhBMP), and a matrix of crushed cancellous allogeneic bone in the clinical
regeneration of functionally useful bone in craniomandibular reconstructions. The history and
current concepts of bone marrow hematopoietic stem cells and mesenchymal stem cells are
reviewed as they relate to bone regeneration in large continuity defects of the mandible.
Materials and Methods: Patients with 6- to 8-cm continuity defects of the mandible with retained
proximal and distal segments were randomized into two groups. Group A received an in situ
tissue-engineered graft containing 54 ± 38 CD34+ cells/mL along with 54 ± 38 CD44+, CD90+,
and CD105+ cells/mL together with rhBMP-2 in an absorbable collagen sponge (1 mg/cm of
defect) and crushed cancellous allogeneic bone. Group B received the same graft, except the
CD34+ cell concentration was 1,012 ± 752 cells/mL. The results were analyzed clinically,
radiographic bone density was measured in Hounsfield units (HU), and specimens were analyzed
histomorphometrically. Results: Forty patients participated (22 men and 12 women; mean age,
57 years). Eight of 20 group A patients (40%) achieved the primary endpoint of mature bone
regeneration, whereas all 20 group B patients (100%) achieved the primary endpoint. CD34+ cell
counts above 200/mL were associated with achievement of the primary endpoint. Bone density
was lower in group A (424 ± 115 HU) than in group B (731 ± 98 HU). Group A bone showed a
mean trabecular bone area of 36% ± 10%, versus 67% ± 13% for group B. Conclusions: The
CD34+ cell functions as a central signaling cell to mesenchymal stem cells and osteoprogenitor
cells in bone regeneration. The mechanism of bone marrow–supported grafts requires a complete
milieu to regenerate large quantities of functionally useful bone. CD34+ cell counts in a
concentration of at least 200/mL in composite grafts are directly correlated to clinically successful
bone regeneration. O
ral
C
raniOfaC
T
issue
e
ng
2012;2:263–271. doi: 10.11607/octe.0059
Key words: bone regeneration, hematopoietic stem cells, mesenchymal stem cells,
recombinant human bone morphogenetic protein
The link between cells of hematopoietic lineage
and marrow osteoprogenitor cells is not new. As
early as 1763, Albrecht von Haller stated that
“the origin of bone is the artery carrying blood and
in it the mineral content.”1 Additionally, the Conhein
hypothesis of 1867 stated that the bloodstream and
consequently the bone marrow was the source of
cells involved in wound regeneration.2 These early
works established the importance of the vascular sys-
tem and bone marrow, along with their associated cell
1 Professor of Surgery and Chief, Division of Oral and
Maxillofacial Surgery, University of Miami Miller School of
Medicine, Miami, Florida, USA.
2 Director of Cellular Science and Education, Harvest Terumo,
Plymouth, Massachusetts, USA.
Correspondence to: Dr Robert E. Marx, University of Miami
Miller School of Medicine, 9380 SW 150th Street, Suite 190,
Miami, FL 33176, USA. Email: rmarx@med.miami.edu
©2012 by Quintessence Publishing Co Inc.
© 2013 BY QUINTESSENCE PUBLISHING CO, INC. PRINTING OF THIS DOCUMENT IS RESTRICTED TO PERSONAL USE ONLY.
NO PART MAY BE REPRODUCED OR TRANSMITTED IN ANY FORM WITHOUT WRITTEN PERMISSION FROM THE PUBLISHER.
© 2013 BY QUINTESSENCE PUBLISHING CO, INC. PRINTING OF THIS DOCUMENT IS RESTRICTED TO PERSONAL USE ONLY.
NO PART MAY BE REPRODUCED OR TRANSMITTED IN ANY FORM WITHOUT WRITTEN PERMISSION FROM THE PUBLISHER.
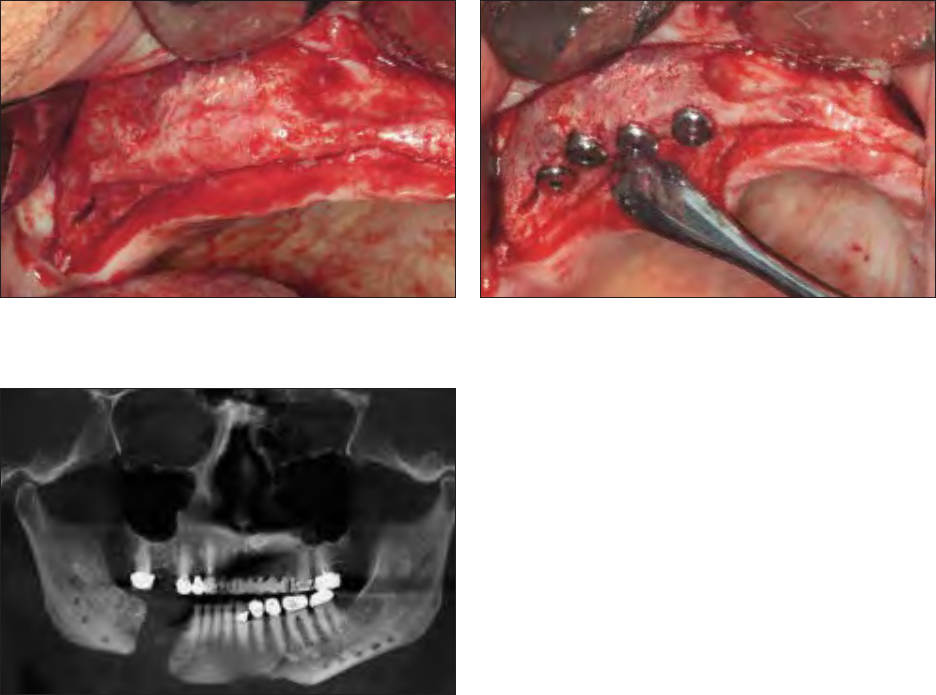
Marx/Harrell
264 Volume 2, Number 4, 2012
lines, in the activation of resident cells within bone for
the purpose of bone regeneration and resident cells
within soft tissue for wound healing.
The current understanding of the cellular composi-
tion of bone marrow aspirates (BMA) and bone mar-
row aspirate concentrates (BMAC) used in today’s
craniofacial bone regeneration involves both hemato-
poietic stem cells (HSCs) and mesenchymal (stromal)
stem cells (MSCs). However, the term stem cells cre-
ates confusion in and of itself, as the term is often used
to refer to both true stem cells as well as to progenitor
cells.3,4 This confusion is multiplied by the common
belief that an MSC represents a pluripotent stem cell
and is all that is required for tissue regeneration,5 a
belief that has not been validated to date.6,7 However,
it has been demonstrated that HSCs are directly in-
volved in both bone regeneration and soft tissue re-
generation,8–10 theoretically supporting the concept
of a pluripotent stem cell to regenerate the entire tis-
sue complex. On the other hand, two landmark pa-
pers11,12 (published in 2005 and 2011, respectively)
identified the paracrine cellular communications and
cell contact between osteoblasts and hematopoietic
stem cell/progenitor cells, suggesting that a multi-
cellular mechanism of MSCs in BMA/BMAC is re-
quired for bone regeneration.
Marx and Tursun identified four important subsets
of stem cells in blood and in BMA (CD34+, CD44+,
CD90+, and CD105+) and found by polymerase
chain reaction and colony-forming unit (CFU) analysis
that their native amounts in the anterior and posterior
ilium were equal and were more than twice that of the
tibial plateau.13 This was followed by a randomized
clinical study, which demonstrated useful and durable
bone regeneration in maxillary alveolar defects of small
volume when combined with platelet-rich plasma,
which contains only small numbers of these MSCs/
progenitor cells, and recombinant human bone mor-
phogenetic protein (rhBMP) (Figs 1 and 2).14 Although
these circulating stem cells/osteoprogenitor cells
proved to be adequate in these smaller defects with
abundant host MSCs/osteoprogenitor cells, they were
found to be incapable of large-volume bone regenera-
tion in continuity defects, where few (if any) host site
resident stem cells or osteoprogenitor cells exist (Fig 3).
Therefore, it became apparent that large continuity
Fig 3 Low numbers of circulating stem cells, even when com-
bined with rhBMP and cancellous allogeneic bone, will not pre-
dictably regenerate bone in large mandibular continuity defects.
Fig 1 Small-volume alveolar ridge bone regeneration was ac-
complished with platelet-rich plasma, rhBMP, and cancellous al-
logeneic bone.
Fig 2 Graft seen in Fig 1; it was capable of receiving dental im-
plants without further augmentation.
© 2013 BY QUINTESSENCE PUBLISHING CO, INC. PRINTING OF THIS DOCUMENT IS RESTRICTED TO PERSONAL USE ONLY.
NO PART MAY BE REPRODUCED OR TRANSMITTED IN ANY FORM WITHOUT WRITTEN PERMISSION FROM THE PUBLISHER.
© 2013 BY QUINTESSENCE PUBLISHING CO, INC. PRINTING OF THIS DOCUMENT IS RESTRICTED TO PERSONAL USE ONLY.
NO PART MAY BE REPRODUCED OR TRANSMITTED IN ANY FORM WITHOUT WRITTEN PERMISSION FROM THE PUBLISHER.
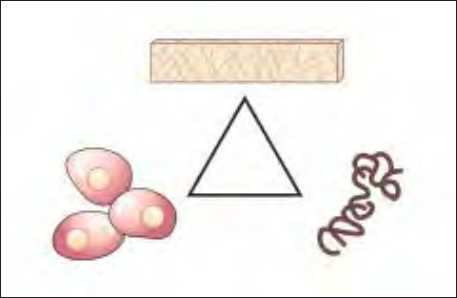
Marx/Harrell
265Oral & Craniofacial Tissue Engineering
defects of the jaws required (1) identification of the
crucial cells in bone marrow that control bone regen-
eration and (2) a means of increasing their numbers.
Several studies have implied that bone marrow
CD34+ cells might be the pivotal cells controlling bone
regeneration. The CD34+ cell has been considered an
HSC.9,15–17 However, studies in the early 2000s iden-
tified it as a cell capable of plasticity18; ie, capable of
differentiation between MSC and HSC lineages.19,20
This concept reinforced the notion that a single cell line
can differentiate into the full cellular tissue composite of
bone, blood vessels, lymphatic tissue, endosteum, peri-
osteum, and bone marrow once again.5 Experiments by
Matsumoto et al confirmed the multilineage differentia-
tion of circulating CD34+ cells, resulting in endothelial
cells for vasculogenesis and osteoblasts for bone regen-
eration.8 This finding was confirmed by others,3 proving
that clonal cells of HSC isolated from bone marrow pro-
duce CFU-fibroblasts (CFU-F) and fibrocytes that were
not of MSC origin but of HSC origin.21,22
The issue that remains under debate is whether
the bone marrow–derived CD34+ cell controls bone
regeneration via its own multilineage differentiation,
followed by expansion or by paracrine and autocrine
signaling to other bone marrow precursor cells and
even by signaling to its parents or progeny to create a
multilineage differentiation and expansion to regener-
ate bone that then undergoes remodeling and renewal.
To explore this issue, the present study used two
concentrations of bone marrow–aspirated CD34+
cells in a proven in situ tissue engineering model.14 This
model combines the selected CD34+ concentration
and bone marrow osteoprogenitor cells with rhBMP-2/
absorbable collagen sponge (rhBMP-2/ACS) and a
matrix of crushed cancellous allogeneic bone, thus com-
pleting the classic tissue-engineering triangle (Fig 4).
MATERIALS AND METHODS
With approval from the institutional review board, pa-
tients with 6- to 8-cm continuity defects of the mandible
were randomized to receive treatment with one of two
protocols. Group A patients received (1) BMA con-
taining total nucleated cells (TNC) 15.5 ± 10
6
/mL and
54 ± 38 cells/mL CD34+ CFU-F; (2) rhBMP-2/ACS,
1 mg/cm of defect; (3) crushed cancellous allogeneic
bone (University of Miami Tissue Bank); and (4) BMA
containing CD44+, CD90+, and CD105+ osteopro-
genitor cells 15.5 × 10
6
TNC and 54 ± 38 cells/mL
CFU-F of each. Group B patients received the same
materials, except that the CD34+ BMA contained
TNC 98 ± 32 × 10
6
/mL and 1,012 ± 752 cells/mL
CD34+ CFU-F.
Method of Bone Marrow Harvest and
Concentration
A total of 60 mL of autologous bone marrow was
harvested from each of four puncture sites in the
bilateral anterior iliac crest intraoperatively at the
point of care using a heparinized trocar to aspirate
15 mL from each site, per the protocol of Marx and
Stevens.
23
Each 60-mL marrow harvest was placed
into a blood transfer bag into which 4 mL of anti-
coagulant citrate dextrose-A had been placed. In
group A patients, 10 mL of this anticoagulated
BMA was used as the CD34+ cellular leg and
the CD44+, CD90+, CD105+ cellular legs of the
tissue-engineering triangle, with TNC counts and
CFU-F cell counts as noted previously. In group
B patients, 60 mL of BMA was processed using a
Smart Prep2 BMAC system (Harvest), which uses
a gradient density centrifugation to provide 10 mL
of CD34+ rich BMAC with the TNC and CFU-F cell
counts as noted earlier.
Patients
Inclusion criteria for the study were:
1. Age over 18 years
2. Radiographic evidence of healed proximal and
distal bone segments in good alignment
3. Sufficient soft tissue to cover a graft without the
need for a local or distant flap
Exclusion criteria were as follows:
1. Previous procedure entering bone marrow
2. History or presence of bone marrow pathology
3. Hypersensitivity to heparin, BMP, or anticoagulant
citrate dextrose-A
4. Previous radiation to the jaws
5. Metastatic cancer
Fig 4 The classic tissue-engineering triangle for predictable tis-
sue regeneration: cells, signal, and matrix.
Matrix
Cells Signal
© 2013 BY QUINTESSENCE PUBLISHING CO, INC. PRINTING OF THIS DOCUMENT IS RESTRICTED TO PERSONAL USE ONLY.
NO PART MAY BE REPRODUCED OR TRANSMITTED IN ANY FORM WITHOUT WRITTEN PERMISSION FROM THE PUBLISHER.
© 2013 BY QUINTESSENCE PUBLISHING CO, INC. PRINTING OF THIS DOCUMENT IS RESTRICTED TO PERSONAL USE ONLY.
NO PART MAY BE REPRODUCED OR TRANSMITTED IN ANY FORM WITHOUT WRITTEN PERMISSION FROM THE PUBLISHER.
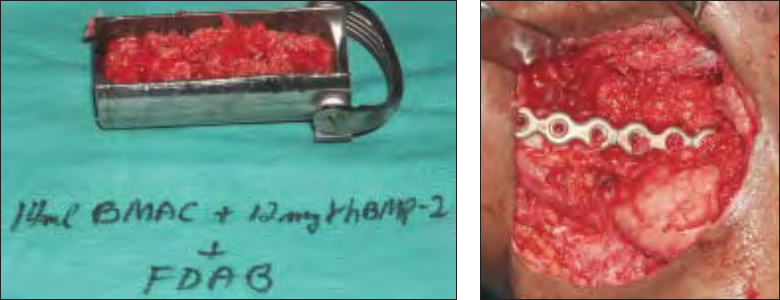
Marx/Harrell
266 Volume 2, Number 4, 2012
6. Exposure to an oral bisphosphonate less than
9 months previous
7. Current regimen or history of receiving an intra-
venous bisphosphonate, methotrexate, or pred ni-
sone
Surgical Procedure
Each patient’s continuity defect was exposed through
a submandibular incision. The recipient tissue bed
was developed by removing scar tissue and reflecting
a minimum of 4 cm of periosteum from both the proxi-
mal and distal segments and scoring the surface edge
of each bone segment to remove the cortex.
The graft was prepared by solubilizing the rhBMP-2
for 5 minutes according to the manufacturer’s direc-
tions (Infuse Bone Graft, Medtronics) and applying it
to the ACS. The rhBMP-2 was allowed a minimum of
15 minutes to bind to the ACS to achieve 95% binding.
Cubes of crushed cancellous allogeneic bone (Uni-
versity of Miami Tissue Bank) were passed through
a bone mill (Stryker Corp) one time using 10 mL
of premilled bone per centimeter of mandibular defect.
The respective anticoagulated BMA or bone marrow
concentrate was added to the milled crushed cancel-
lous allogeneic bone and mixed thoroughly. Then the
saturated rhBMP-2/ACS was cut into 1-cm square
pieces and added to the mixture to create a compos-
ite graft. Prior to placement, 0.5 mL of a 10% cal-
cium chloride solution containing 5,000 IU of bovine
thrombin was added to the composite graft to reverse
the anticoagulant, release the growth factors inherent
in the platelet fraction, and activate the cell adhesion
molecules in the plasma fraction.
This composite graft (Fig 5) was placed and con-
densed into the prepared continuity defect using Pen-
field bone packers (Fig 6). The graft was contained
with either a titanium mesh or an allogeneic bone strut,
as appropriate to the morphology of the defect. Four
weeks of maxillomandibular fixation followed for each
patient, along with 1 week of postoperative antibiotics
and appropriate analgesics.
Assessments and Follow-up
All patients underwent examination and release of
maxillomandibular fixation and arch bar removal under
local anesthesia at 4 weeks. A baseline cone beam
computed tomographic (CT) scan was taken at that
time. Examinations and cone beam CT scans were
also performed at 3 and 6 months. At 6 months, the
principal investigator determined whether there was
sufficient bone and sufficient mineral density to ac-
commodate dental implants (primary endpoint). Those
patients in whom the clinical and radiographic assess-
ments indicated implant placement received implants
(Biomet 3i), and a core or open bone biopsy specimen
was harvested. All implants were allowed 6 months for
osseointegration before functional loading.
Study Endpoints
The primary endpoint was sufficient bone to place dental
implants without the need for additional grafting. Patients
who regenerated sufficiently dense bone capable of im-
plant primary stability were considered responders. One
secondary endpoint was the radiographic density of the
bone in Hounsfield units (HU) taken from the 6-month
cone beam CT scan. Another secondary endpoint was
the analysis of trabecular bone density obtained from
histomorphometric analysis of the bone cores or biopsy
specimens taken at implant placement using the Image-
Pro Plus 5.0 computer-based analyzer.
Statistical Analysis
Data evaluation was accomplished using the sta-
tistical software package SPSS 13.0 (SPSS Inc).
Discrete variables were presented as cell counts and
percentages. A paired t test was used to analyze the
Fig 5 Composite bone graft of BMAC (CD34+ cell–enriched),
rhBMP, and cancellous allogeneic bone.
Fig 6 At surgery, the composite graft
shows a loose consistency.
© 2013 BY QUINTESSENCE PUBLISHING CO, INC. PRINTING OF THIS DOCUMENT IS RESTRICTED TO PERSONAL USE ONLY.
NO PART MAY BE REPRODUCED OR TRANSMITTED IN ANY FORM WITHOUT WRITTEN PERMISSION FROM THE PUBLISHER.
© 2013 BY QUINTESSENCE PUBLISHING CO, INC. PRINTING OF THIS DOCUMENT IS RESTRICTED TO PERSONAL USE ONLY.
NO PART MAY BE REPRODUCED OR TRANSMITTED IN ANY FORM WITHOUT WRITTEN PERMISSION FROM THE PUBLISHER.

Marx/Harrell
267Oral & Craniofacial Tissue Engineering
BMA and BMAC cell counts. A multivariate logistic re-
gression analysis was used to study predictors of clin-
ical benefit after BMA and BMAC graft applications.
For all analyses, P < .05 was considered significant.
RESULTS
Forty patients (mean age, 57 years; range, 19 to 78
years; 22 men, 12 women) participated in the study.
All patients proceeded through the postoperative
course without significant complications and showed
evidence of new bone regeneration by 6 months.
Table 1 identifies achievement of the primary end-
point in each group. Eight of 20 group A patients
(40%) achieved the primary endpoint (Figs 7 and 8),
whereas all 20 group B patients (100%) achieved the
primary endpoint (Figs 9 and 10); this difference was
statistically significant (P = .006). This correlates to a
CD34+ cell count of 54 ± 38 cells/mL in group A ver-
sus a CD34+, cell count of 1,012 ± 752 cells/mL in
group B, while the concentrations of progenitor cells
of CD44+, CD90+ and CD 105+ were nearly equal
between the two groups.
According to univariate analysis, a CD34+ cell count
of 200/mL CFU-F was associated with a clinical out-
come of sufficient bone regeneration to accommodate
Fig 7 Group A graft that did not achieve the primary endpoint. Bone height, quan-
tity, and maturity were inadequate at 6 months.
Fig 8 Group A graft cone beam CT slice in-
dicating bone regeneration with voids and the
absence of a cortical rim.
Fig 9 Group B graft that met the primary endpoint. Bone height, quan-
tity, and maturity are present at 6 months.
Fig 10 Group B graft cone beam
CT slice indicating complete bone
regeneration with a cortical rim and
trabecular bone without voids.
Table 1 Results of Treatment
Endpoint
Group A
(n = 20)
Group B
(n = 20) P
Regeneration of
implantable bone
8/20 (40%) 20/20 (100%) .006
Mean radiographic
density
424 ± 115 HU 731 ± 98 HU .01
Mean trabecular
bone area
36% ± 10% 67% ± 13% .01
© 2013 BY QUINTESSENCE PUBLISHING CO, INC. PRINTING OF THIS DOCUMENT IS RESTRICTED TO PERSONAL USE ONLY.
NO PART MAY BE REPRODUCED OR TRANSMITTED IN ANY FORM WITHOUT WRITTEN PERMISSION FROM THE PUBLISHER.
© 2013 BY QUINTESSENCE PUBLISHING CO, INC. PRINTING OF THIS DOCUMENT IS RESTRICTED TO PERSONAL USE ONLY.
NO PART MAY BE REPRODUCED OR TRANSMITTED IN ANY FORM WITHOUT WRITTEN PERMISSION FROM THE PUBLISHER.
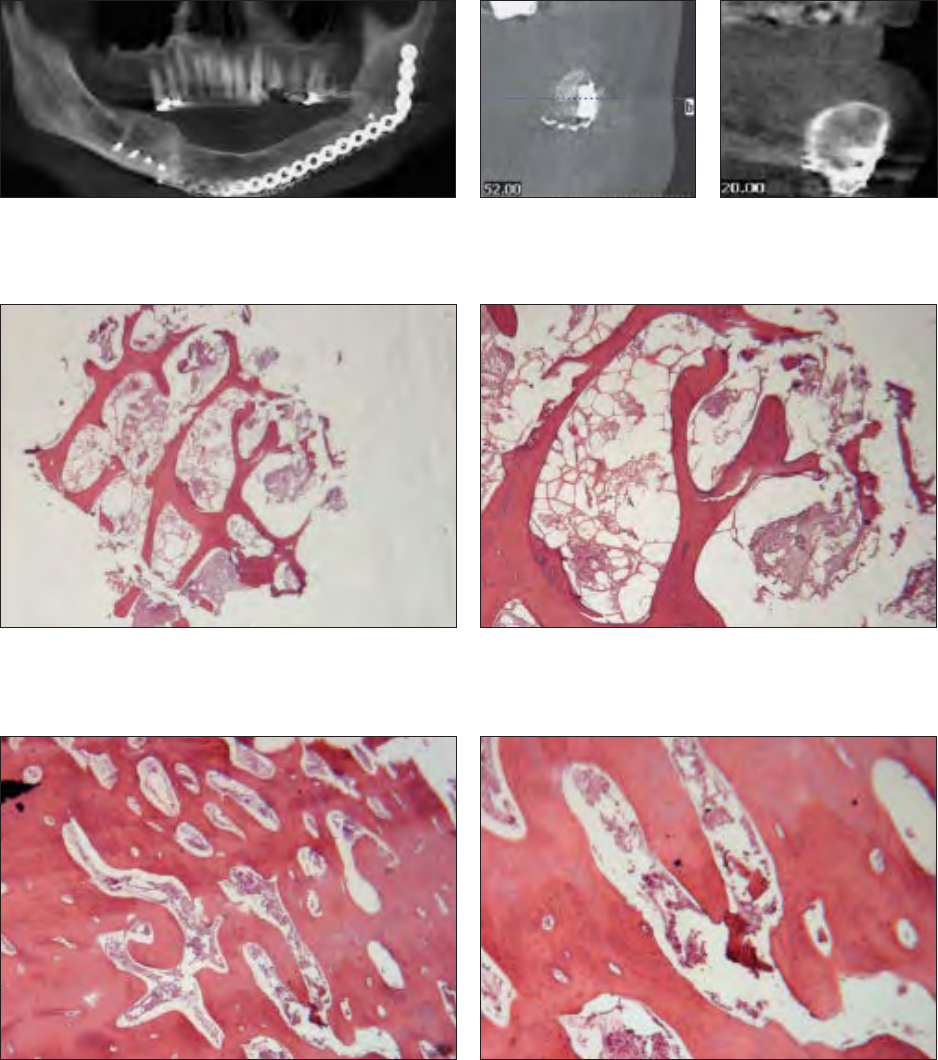
Marx/Harrell
268 Volume 2, Number 4, 2012
dental implant placement and gain primary stability
without the need to augment the regenerated bone
(P = .011, odds ratio 5.1, 95% confidence interval
1.25 to 20.52) (Fig 11).
Table 1 also illustrates the mean radiographic den-
sity of the regenerated bone in both groups. Group
A sites showed only 58% of the density of group B
sites (424 ± 115 HU and 731 ± 98 HU, respectively)
(Figs 12 and 13) (P = .01). The trabecular bone
area of group A (36% ± 10%) was only 54% of the
group B trabecular bone area (67% ± 13%) (Figs 14
to 17) (P = .01).
Fig 14 Group A graft with trabecular bone area of 28%. Note the
thin trabecular bone struts and the fibrous-fatty marrow spaces that
occupy much of the volume instead of bone (hematoxylin-eosin;
original magnification ×1.6).
Fig 16 Group B graft with trabecular bone area of 74%. Note
the thick bone trabecular struts and regeneration of marrow cells
rather than fibrous-fatty marrow (hematoxylin-eosin; original mag-
nification ×1.6).
Fig 15 Group A graft at higher power. Mature bone with lamellar
architecture but insufficient quantity and thin trabecular struts is
present; haversian canals are absent (hematoxylin-eosin; original
magnification ×10).
Fig 17 Group B graft at higher power. Mature bone shows la-
mellar architecture, small cellular marrow spaces, and haversian
canals (hematoxylin-eosin; original magnification ×10).
Fig 12 Radiographic bone
density of a group A graft
(512 HU).
Fig 13 Radiographic bone
density of a group B graft
(794 HU).
Fig 11 Complete bone regeneration occurs if the CD34+ cell
count exceeds 200/mL. Here, a CD34+ cell count of 292/mL
provided the required crucial cell count in the tissue-engineering
triangle.
© 2013 BY QUINTESSENCE PUBLISHING CO, INC. PRINTING OF THIS DOCUMENT IS RESTRICTED TO PERSONAL USE ONLY.
NO PART MAY BE REPRODUCED OR TRANSMITTED IN ANY FORM WITHOUT WRITTEN PERMISSION FROM THE PUBLISHER.
© 2013 BY QUINTESSENCE PUBLISHING CO, INC. PRINTING OF THIS DOCUMENT IS RESTRICTED TO PERSONAL USE ONLY.
NO PART MAY BE REPRODUCED OR TRANSMITTED IN ANY FORM WITHOUT WRITTEN PERMISSION FROM THE PUBLISHER.
Marx/Harrell
269Oral & Craniofacial Tissue Engineering
DISCUSSION
This study investigated the role of the bone marrow–
derived CD34+ cell in a milieu of osteoprogenitor
cells, bone marrow plasma cell adhesion molecules,
rhBMP, and a matrix of crushed cancellous allogeneic
bone in the clinical regeneration of functionally useful
bone in craniomandibular reconstructions. The main
findings can be summarized as follows:
1. The CD34+ cell as an HSC is the crucial cell
among the other stem cells/osteoprogenitor cells
in regenerating bone in an in situ human tissue-
engineering model.
2. A CD34+ cell count of 200/mL CFU-F or greater is
directly correlated to a successful clinical outcome.
3. The correlation of extensive bone regeneration
with higher counts of CD34+ cells as compared
to lower counts of CD34+ cells together with
equal counts of other MSC/osteoprogenitor cells
and cell adhesion molecules in the milieu strong-
ly supports the mechanism of action proposed
by others; that is, a master signaling cell using
paracrine and autocrine cellular cross talk to up-
regulate other bone marrow stem cells/progenitor
cells of either HSC or MSC origin, resulting in sig-
nificant bone regeneration within the environment
of the graft.12,24–26
CLINICAL IMPLICATIONS
This study demonstrates that BMA by itself is in-
sufficient to predictably regenerate clinically useful
bone in large bony continuity defects of the man-
dible. Concentrations of bone marrow to achieve a
CD34+ cell count of at least 200 CFU-F/mL are
necessary to provide predictable bone regeneration
in the context of the composite graft system used in
this study. Therefore, devices must be capable of con-
centrating anticoagulated bone marrow to five to eight
times baseline levels and to particularly focus on the
CD34+ cell population while still retaining baseline
levels of CD44+, CD90+, and CD105+ cells.27
Improved Understanding of Adult
Bone Marrow Stem Cells
Beyond using the results of this stem cell study to im-
prove clinical outcomes, this study also sheds some
light on the understanding of cell-to-cell, cell-to-
matrix, and cell-to-microenvironment interactions
within human adult bone marrow that may result in fur-
ther improvements in clinical outcomes.
Although hematopoeisis was once thought to be the
sole function of bone marrow,28 work by Friedenstein
et al in 1966 identified bone marrow MSCs other than
HSCs, thereby defining two distinct classes of multi-
potent human bone marrow stem cells: HSCs and
MSCs.29 They isolated CFU-F multipotent cells from
marrow plastic adherent cells and found that they could
differentiate into osteoblasts, chondrocytes, adipocytes,
and supporting stroma for hematopoietic cells. Hence,
they have often been referred to as mesenchymal stro-
mal cells as well as mesenchymal stem cells.
HSCs were originally thought to solely produce the
lineage of red and white blood cells and thrombocytes/
platelets and reconstitute the bone marrow stem cell
population. However, more recent studies have identi-
fied that HSCs are significantly plastic, producing and
up-regulating MSCs and their progeny, and are not
limited to blood cell production.20,30,31 Researchers
now realize that these two classes of stem cells are
not independent of each other but are actually very in-
terdependent.31,32 Furthermore, each is influenced by
its own anatomical niche,10,11,33 various growth fac-
tors,25,34 and by the local microenvironment into which
it may be placed.35
In the context of this study, the HSCs were rep-
resented by the CD34+ cell and the MSCs by the
CD44+, CD90+, and CD105+ cells. Each cell
responded to the peripheral signals in the micro-
environment, represented by the rhBMP-2 as well as
the inherent hypoxia in the wound, the growth factors
from the platelets and macrophages, and the cell ad-
hesion molecules present throughout the graft. The
subsequent cellular proliferations were directed by
the CD34+ cell, and the osteoblast differentiation
and osteoid synthesis were directed, up-regulated,
and stimulated by all of the cellular components,
growth factors, and matrix proteins in the graft. These
cell-to-cell, cell-to-matrix, and growth-factor-to-cell
interactions resulted in the useful bone regeneration
observed and emphasize the importance of cellular
heterogeneity in graft systems.
Although significant research efforts have been
made to identify one specific cell type or one specific
growth factor that would significantly increase tissue
regeneration, this study and others demonstrate that
a heterogenous population of HSCs and MSCs, de-
livered with numerous but specific growth factors in
a conducive microenvironment, provides more pre-
dictable bone regeneration. The importance and sup-
portive actions of the various cellular components in
human bone marrow are outlined in Table 2, and the
supportive actions of growth factors and the microen-
vironment are outlined in Table 3. Although the CD34+
cell is a crucial and perhaps outcome-determining
cell, it does not act alone and cannot regenerate bone
without a diversity of cells in the milieu and the various
signals from growth factors and the microenvironment.
© 2013 BY QUINTESSENCE PUBLISHING CO, INC. PRINTING OF THIS DOCUMENT IS RESTRICTED TO PERSONAL USE ONLY.
NO PART MAY BE REPRODUCED OR TRANSMITTED IN ANY FORM WITHOUT WRITTEN PERMISSION FROM THE PUBLISHER.
© 2013 BY QUINTESSENCE PUBLISHING CO, INC. PRINTING OF THIS DOCUMENT IS RESTRICTED TO PERSONAL USE ONLY.
NO PART MAY BE REPRODUCED OR TRANSMITTED IN ANY FORM WITHOUT WRITTEN PERMISSION FROM THE PUBLISHER.
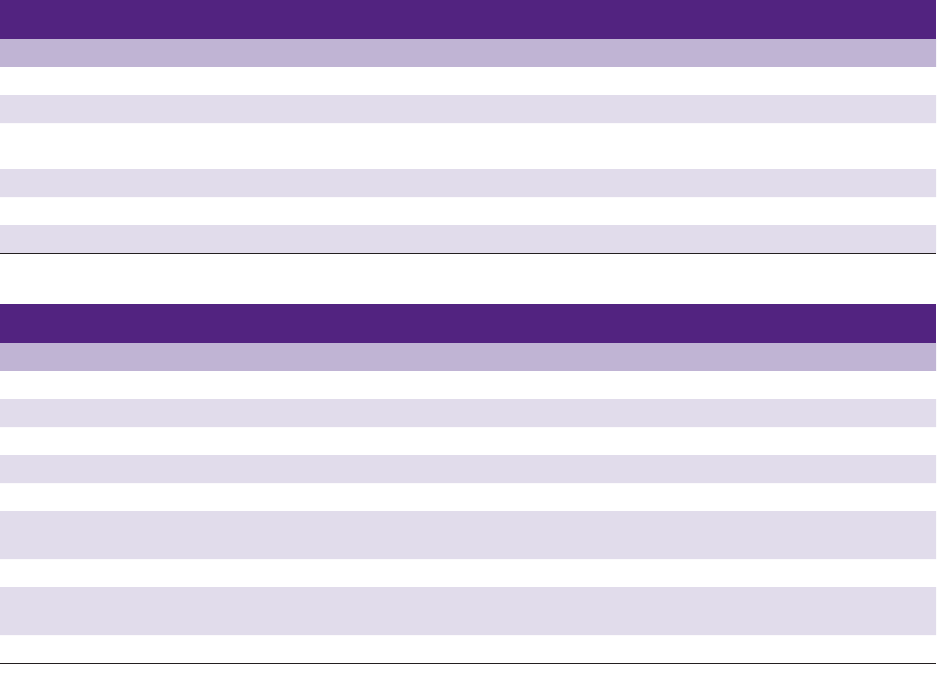
Marx/Harrell
270 Volume 2, Number 4, 2012
Therefore, concentrating the cellular components
of whole bone marrow, rather than isolating and ex-
panding selected MSCs and/or HSCs, optimizes and
promotes both osteogenesis and angiogenesis. The
authors suggest that the use of the full diversity of hu-
man nucleated bone marrow cells and increasing their
numbers translates into enhanced bone regeneration
that is superior to outcomes obtained by single cell
expansions or single growth factor applications.
The long-awaited goal of rebuilding lost skeletal
parts of significant size without the morbidity of open
bone harvesting (eg, a long hospital stay, a long con-
valescent period, scarring, disability, and higher costs)
has now become a reality. Although researchers have
just begun to understand the complexities of human
bone marrow cell functions and their interactions, the
value of rhBMPs, and the matrices upon which bone
can regenerate, it is known that composite grafts of
an appropriate cellular milieu, a signal, and a suitable
matrix can regenerate up to 8 cm of missing bone. The
challenge now is to expand the knowledge and un-
derstanding of adult bone marrow cells, the signal of
rhBMP, and the best matrix for bone growth to apply
these results to larger skeletal defects and those as-
sociated with a hostile tissue environment (eg, radiat-
ed tissue, scar tissue, bisphosphonate-compromised
bone).
ACKNOWLEDGMENTS
Dr Marx is a paid consultant for Harvest Technologies and
Medtronic, Inc. Dr Harrell is the chief research scientist at Harvest
Technologies.
REFERENCES
1. Trueta J. The role of the vessels in osteogenesis. J Bone Joint
Surg 1963;45B:402–418.
2. Wohlrab F, Henoch U. [The life and work of Carl Weigart
(1845–1904) in Leipzig 1878–1885.] Zentralb Allg Pathol
1988;134:743–751.
3. Caplan AL. What’s in a name? Tissue Eng Part A 2010;16:
2415–2417.
Table 3 Activities and Roles of the Growth Factors and the Microenvironment in a Graft
Factor Action
Hypoxia Driving force for wound healing via macrophage chemotaxis
Fibronectin and fibrin (from plasma) Cell adhesion molecules; matrix for osteosynthesis
Vitronectin (from platelets) Cell adhesion molecule; matrix for osteosynthesis
Stromal-derived activating factor 1-alpha (from platelets) Homing signal for MSCs and HSCs
Platelet-derived growth factors aa, ab, bb (from platelets) Protein isomers that are mitogenic and angiogenic
Transforming growth factor beta-1 and beta-2 (from platelets) Protein isomers that are mitogenic, angiogenic, and
support MSC differentiation toward bone and cartilage
Vascular endothelial growth factor (from platelets and macrophages) Angiogenesis
BMP Attraction for MSCs and HSCs; proliferation and
osteoblast differentiation; stimulation of osteoid synthesis
Macrophages Secretion of growth factors for completion of wound healing
Table 2 Activity of Various Cellular Components of Human Whole Bone Marrow
Component Role
MSC Differentiate into osteoblasts; bone formation; respond to HSCs and growth factors
HSC Drive regeneration via angiogenesis/vasculogenesis; orchestrate bone formation; convert to MSC “plasticity”
Endothelial
progenitor cells
Stimulate angiogenesis; release BMP-2 and BMP-6; up-regulate BMP-2 production
Platelets Mediate cell-to-cell adhesion and cell-to-matrix adhesion via cell adhesion molecules; release growth factors
Lymphocytes Support migration and proliferation of endothelial progenitor cells
Granulocytes Release vascular endothelial growth factor to support angiogenesis and vasculogenesis
© 2013 BY QUINTESSENCE PUBLISHING CO, INC. PRINTING OF THIS DOCUMENT IS RESTRICTED TO PERSONAL USE ONLY.
NO PART MAY BE REPRODUCED OR TRANSMITTED IN ANY FORM WITHOUT WRITTEN PERMISSION FROM THE PUBLISHER.
© 2013 BY QUINTESSENCE PUBLISHING CO, INC. PRINTING OF THIS DOCUMENT IS RESTRICTED TO PERSONAL USE ONLY.
NO PART MAY BE REPRODUCED OR TRANSMITTED IN ANY FORM WITHOUT WRITTEN PERMISSION FROM THE PUBLISHER.
Marx/Harrell
271Oral & Craniofacial Tissue Engineering
4. Modder UL, Koshla S. Skeletal stem/osteoprogenitor cells:
Current concepts, alternate hypotheses, and relationship to
the bone remodeling compartment. J Cell Biochem 2008;
103:393–400.
5. Krause DS, Theise ND, Collector MI, et al. Multiorgan,
multi-lineage engraftment by a single bone marrow-derived
stem cell. Cell 2001;105:369–377.
6. Dominici M, Pritchard C, Garlits JE, Hoffman TJ, Persons DA,
Horwitz EM. Hematopoietic cells and osteoblasts are derived
from a common marrow progenitor after bone marrow
transplantation. Proc Nat Acad Sci U S A 2004;101:
11761–11766 .
7. Chen JL, Hunt P, McElvain M, Black T, Kaufman S, Choi ES.
Osteoblast precursor cells are found in CD34+ cells from
human bone marrow. Stem Cells 1997;15:368–377.
8. Matsumoto T, Kuroda R, Mifune Y, et al. Circulating endothe-
lial/skeletal progenitor cells for bone regeneration and
healing. Bone 2005;43:434–439.
9. Matsumoto T, Kawamoto A, Kuroda R, et al. Therapeutic
potential of vasculogeneis and osteogenesis promoted by
peripheral blood CD34 positive cells for functional bone
healing. Am J Pathol 2006;167:1440–1457.
10. Taichman RS. Blood and bone: Two tissues whose fates are
intertwined to create the hematopoietic stem cell niche. Blood
2005 Apr 1;105(7):2631–2639. Epub 2004 Dec 7.
11. Lo Celso C, Scadden DT. The hematopoietic stem cell niche
at a glance. J Cell Sci 2011;124:3529–3535.
12. Jung Y, Wang J, Havens A, Sun Y, Jin T, Taichman RS. Cell to
cell contact is critical for the survival of hematopoietic
progenitor cells on osteoblasts. Cytokine 2005;32:155–162.
13. Marx RE, Tursun R. A qualitative and quantitative analysis of
autologous human multipotent adult stem cells derived from
three anatomic sites by marrow aspiration: Tibia, anterior ilium
and posterior ilium. Oral Craniofac Tissue Eng 2011;1:98–102.
14. Marx RE, Armentano L, Olavarria A, Samaniego J. rhBMP-2/
ACS versus autogenous cancellous marrow grafts in large
vertical defects of the maxilla: An unsponsored randomized
open label clinical trial. Oral Craniofac Tissue Eng 2011;1:
33–41.
15. Turan RG, Bozdag-Turan I, Ortak J, et al. Impaired mobiliza-
tion of CD 133+ bone marrow derived circulating progenitor
cells with an increased number of diseased coronary arteries
in ischemic heart disease patients with diabetes. Circ J 2011;
75:2635–2641.
16. Asahara T, Murohara T, Sullivan A, et al. Isolation of putative
progenitor endothelial cells form angiogenesis. Science
1997;275:964–967.
17. Asahara T, Musuda H, Takalashi T, et al. Bone marrow origin
of endothelial progenitor cells responsible for post natal
vasculogenesis in physiological and pathological meovascu-
larizaiton. Circ Res 1999;85:221–228.
18. Forbes SJ, Viz P, Poulsom R, Wright NA, Absen MR. Adult
stem cell plasticity: New pathways of tissue regeneration
become visible. Clin Sci 2002;103:355–369.
19. Grove JE, Bruscia E, Krause DS. Plasticity of bone marrow
derived stem cells. Stem Cells 2004;22:487–500.
20. Lakskmipathy U, Verfaille C. Stem cell plasticity. Blood Rev
2005;19:29–38.
21. Larue AG, Masuya M, Ebihara Y, et al. Hematopoietic origins
of fibroblasts I. In vivo studies of fibroblasts associated with
solid tumors. Exp Hematol 2006;34:208–218.
22. Ebihara Y, Masuya M, Larue AC, et al. Hematopoietic origins
of fibroblasts II. In vitro studies of fibroblasts, CFU-F, and
fibrocytes. Exp Hematol 2006;34:219–229.
23. Marx RE, Stevens MR (eds). Atlas of Bone Harvesting.
Hanover Park, IL: Quintessence, 2010:141–149.
24. Yasuhara S, Yasunaga Y, Hisatome T, et al. Efficacy of bone
marrow mononuclear cells to promote bone regeneration
compared with isolated CD34+ cells from the same volume
of aspirate. Artif Org 2010;34:594–599.
25. Nakajima H. Role of transcription factors in differentiation and
reprogramming of hematopoietic cells. New Engl J Med 2011;
60:47–55.
26. Tash D, Slack JM. How cells change their phenotype. Nat Rev
Mol Cell Biol 2002;3:187–194.
27. Hermann PC, Huber SL, Herrler T, et al. Concentration of
bone marrow total nucleated cells by a point of care device
provides a high yield and preserves their functional activity.
Cell Transplant 2008;16:1059–1069.
28. Yoder MC. Overview of stem cells biology. In: Hoffman R,
Beng EJJ, Shattil SS, et al (eds). Hematology: Basic
Principles and Practice, ed 5. Philadelphia, PA: Churchill
Livingstone Elsevier, 2009:187–199.
29. Friedenstein AJ, Piatetsky S, II, Petrakova KV. Osteogenesis
in transplants of bone marrow cells. J Embryol Exp Morphol
1966;16:381–390.
30. Quesenberry PS, Abedi M, Aliotta JM, et al. Stem cell
plasticity: An overview. Blood Cells Mol Dis 2004;32:1–4.
31. Quesenberry PJ, Donner MS, Aliotta JM. Stem cell plasticity
revisited: The continuum marrow model and phenotypic
changes mediated by microvesicles. Exp Hematol 2010
Jul;38(7):581–592. Epub 2010 Apr 9.
32. Quesenberyy PJ, Aliotta JM. Cellular phenotype switching
and microvesicles. Adv Drug Deliv Rev 2010;62:1141–1148.
33. Scholfield R. The relationship between the spleen colony-
forming cell and the hematopoietic stem cell. Blood Cells
1978;4:7–25.
34. Shaked Y, Tang T, Woloszynek J, et al. Contribution of
granulocyte colony-stimulating factor to the acute mobilization
of endothelial precursor cells by vascular disrupting agents.
Cancer Res 2009;69:7524–7528.
35. Theise ND, d’Inverno M. Understanding cell lineages as
complex adaptive systems. Blood Cells Mol Dis 2004;
32:17–20.
© 2013 BY QUINTESSENCE PUBLISHING CO, INC. PRINTING OF THIS DOCUMENT IS RESTRICTED TO PERSONAL USE ONLY.
NO PART MAY BE REPRODUCED OR TRANSMITTED IN ANY FORM WITHOUT WRITTEN PERMISSION FROM THE PUBLISHER.
© 2013 BY QUINTESSENCE PUBLISHING CO, INC. PRINTING OF THIS DOCUMENT IS RESTRICTED TO PERSONAL USE ONLY.
NO PART MAY BE REPRODUCED OR TRANSMITTED IN ANY FORM WITHOUT WRITTEN PERMISSION FROM THE PUBLISHER.